Jacob used a military analogy to explain the genetic ‘circuit’ he had discovered with Monod, unwittingly returning to Wiener’s original recognition of the role of feedback through his work on anti-aircraft fire control:
We saw this circuit as made up of two genes: transmitter and receiver of a cytoplasmic signal, the repressor. In the absence of the inducer, this circuit blocked the synthesis of galactosidase. Every mutation inactivating one of the genes thus had to result in a constitutive synthesis, much as a transmitter on the ground sends signals to a bomber: ‘Do not drop the bombs. Do not drop the bombs.’ If the transmitter or the receiver is broken, the plane drops its bombs. But let there be two transmitters with two bombers, and the situation changes. The destruction of a single transmitter has no effect, for the other one will continue to emit. The destruction of a receiver, however, will result in dropping the bombs, but only by the bomber whose receiver is broken.
29
A few months later, Monod gave a lecture in Germany and described the model in more precise terms, adopting the vocabulary of information and control: ‘We could imagine that the
z
locus contains genetic information relating to the structure of the galactosidase protein, while the
i
locus determines the conditions under which this information is potentially transferred to the cytoplasm.’ By implication, Monod was arguing that that the ‘one gene, one enzyme’ idea – less than 20 years old – could not explain the complex reality of protein synthesis. Instead, he suggested there were two kinds of genes: structural genes, which contained the information necessary to make proteins, and regulator genes, which determined when that information was employed, by synthesising a specific repressor that inhibited the expression of the structural gene.
30
In March 1959, the final version of the PaJaMo experiment was submitted to a new academic publication, which had a title that was a manifesto for the new science: the
Journal of Molecular Biology.
In the paper, which was more developed than the original French publication, the trio acknowledged that they were ‘much indebted to Professor Leo Szilárd for illuminating discussions’. The paper presented the dense details of their experiment and outlined the repressor hypothesis, suggesting that it was a general model for protein synthesis and showing the parallels with the phage work of François Jacob and Élie Wollman. Above all, they highlighted two points that they could not answer: the nature of the repressor and how it worked.
31
As they explored these issues, Jacob and Monod changed the vocabulary that was used for talking and thinking about genes and what they – and the code they contain – might do.
*
For much of the 1950s, scientists had felt uncomfortable about the word ‘gene’. In 1952, the Glasgow-based Italian geneticist Guido Pontecorvo highlighted the existence of four different definitions of the word that were regularly employed by scientists and which were sometimes mutually contradictory. A gene could refer to a self-replicating part of a chromosome, the smallest part of a chromosome that can show a mutation, the unit of physiological activity or, finally, the earliest definition of a gene – the unit of hereditary transmission.
32
Pontecorvo questioned whether the gene could any longer be seen as a delimited part of a chromosome, and suggested instead that it was better seen as a process and that the word gene should therefore be used solely to describe the unit of physiological action.
Although Pontecorvo’s suggestion was not taken up, scientists recognised the problem. The debate over words and concepts continued at the Johns Hopkins University symposium on ‘The Chemical Basis of Heredity’, which was held in June 1956. By this time it was generally accepted as a working hypothesis that all genes in all organisms were made of DNA and that the Watson–Crick double helix structure was also correct. Joshua Lederberg, a stickler for terminology, declared audaciously that ‘“gene” is no longer a useful term in exact discourse’.
33
He would no doubt be surprised to learn that it is still being used, more than half a century later. At the same meeting, Seymour Benzer came up with a solution:
The classical ‘gene’, which served at once as the unit of genetic recombination, of mutation, and of function, is no longer adequate. These units require separate definition.
34
Since 1954, Benzer had been studying the structure of genes, focusing on the rII genetic region of the T4 phage virus.
35
From today’s perspective, it is noteworthy that at the 1956 meeting Benzer was still hedging his bets over the nature of the genetic material in T4: all he was prepared to say at that point was that in this virus DNA ‘appears to carry the hereditary information’.
36
By screening hundreds of mutants in the rII region of the virus’s DNA and then carrying out thousands of crosses to see whether they were part of the same functional unit, Benzer was able to construct an extremely detailed genetic map, down to a single pair of nucleotides. Like Rutherford showing that the atom had an internal structure through his work in Manchester at the beginning of the twentieth century, Benzer was able to show that the gene was not a single, indivisible unit.
37
Rather than the classic image of the gene as a bead on a string, Benzer saw the gene as a one-dimensional stretch of DNA, which would reveal its secrets by dissecting it down to the molecular level. He found that not all parts of the gene were equal – some areas were much more prone to spontaneous mutations, and mutations in different areas produced different effects. Long before the development of DNA sequencing, Benzer’s pioneering and painstaking study showed that genes have an internal structure.
On the basis of this work, Benzer came up with new words, focusing on what genes actually did: the unit of genetic recombination was a ‘recon’, the smallest unit of mutation was a ‘muton’, and the unit of function was a ‘cistron’.* Although only ‘cistron’ survived into common scientific usage for a while (it is now very much on the wane), Benzer’s attempt to reconceive the gene in molecular terms was highly influential. Widely praised at the time – at the Johns Hopkins symposium, George Beadle described it as ‘very beautiful work’ – Benzer’s approach helped fuse the structural insights of Watson and Crick with the traditional approaches of genetics, creating the new subject of molecular genetics.
38
This new field was reinforced in 1957 by two PhD students, Matthew Meselson and Frank Stahl, who carried out what has been described as ‘the most beautiful experiment in biology’.
39
One of the problems raised by the double helix structure was how the DNA molecule copied itself. The complementarity of the base pairs on the two strands suggested that the cell used each strand as a template to create two identical double helices, but how this worked was unclear. At the same 1956 Johns Hopkins meeting at which Benzer spoke, Max Delbrück outlined three models for DNA replication – ‘conservative’, in which the original DNA double helix remained intact and was entirely copied into a completely new molecule; ‘semi-conservative’, in which one strand of each molecule was copied, producing two daughter molecules, each of which had one old and one new strand (this was the model suggested by Watson and Crick); and Delbrück’s preferred view, ‘dispersive’ replication, whereby bits of each DNA strand were copied and then reassembled, producing two double helices, each strand of which was made up of a mixture of old and new.
40
Meselson and Stahl’s experiment was designed to distinguish between these three hypotheses.
They began planning their experiment in 1954; they decided to use a heavy isotope of nitrogen (
15
N; normal nitrogen has an atomic weight of 14) to distinguish between the original strand and its copy. Nitrogen is an important atomic component of DNA, so DNA that was synthesised with
15
N was slightly heavier than its
14
N counterpart. After three years of preliminary experiments, they grew
E. coli
bacteria for several generations on a medium rich in
15
N, thereby creating a stock of bacteria with
15
N-based DNA. The bacteria were then transferred to medium containing normal
14
N and were allowed to reproduce for several cycles before their DNA was extracted. Meselson and Stahl spun the samples in an ultracentrifuge machine at 44,700 r.p.m. for 20 hours – any double helix containing
14
N ended up at a different position in the tube from its
15
N equivalent, simply because it was lighter.
The results were very clear. After one cycle of reproduction, in which the bacteria had made only one copy of their DNA, two kinds of DNA were detected: a heavy band, composed of the original
15
N-based DNA, and a slightly lighter band composed of a mixture of
15
N and
14
N-based DNA. This showed that ‘conservative’ replication was wrong – that model predicted that only
14
N-based DNA would be present in the newly synthesised molecules. The next step was decisive: if the bacteria were allowed to reproduce for another cycle, then a new, lighter, band composed only of
14
N-based DNA appeared. These molecules must have been created by copying from a whole strand of
14
N DNA that had been created after the first round of replication. This showed that Watson and Crick were right and Delbrück was wrong: DNA replication was ‘semi-conservative’ with, most probably, the whole of each strand being copied simultaneously to make new daughter molecules.
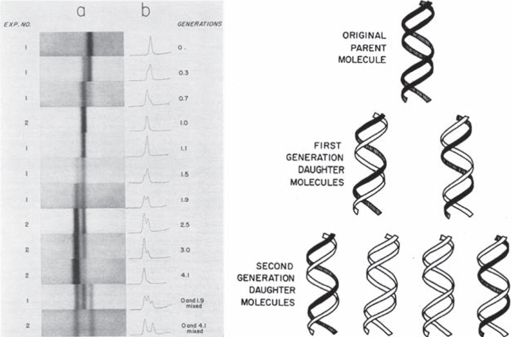
7. Figures from Meselson and Stahl’s 1958 paper. Left: DNA produced by two identical experiments (1 and 2), shown as a band (1) and a graph (r). At the beginning there was only
15
N DNA. As the bacteria reproduced on
14
N-rich culture, two lighter forms appeared, one after one generation, the other after two generations. By generation 4, most of the DNA was of the lightest type. Right: Meselson and Stahl’s interpretation. In each round of reproduction the bacterial DNA was copied, using nitrogen from the
14
N medium. After one generation, each DNA double helix was composed of a new
14
N strand and an old
15
N DNA strand. At the second generation, some molecules were composed entirely of
14
N-rich DNA, and were therefore lighter again.
By resolving the thorny problem of DNA replication, Meselson and Stahl’s elegant and precise experiment represented the final confirmation of the significance of the double helix structure of DNA. As it closed one phase of the history of molecular biology, it opened another, showing that DNA molecules – and hence the genes they contained – could be investigated by using the latest analytical techniques.
Three studies from the late 1950s therefore pointed the way to the future: Meselson and Stahl showed that DNA could be labelled and tracked down the generations, Benzer’s fastidious work revealed that it was possible to investigate the molecular structure of the gene by exploring its tiniest components, and Ingram’s discovery that the sickle-cell mutation in the haemoglobin gene produced a single amino acid change hinted that the nature of the genetic code itself might be within reach.
*
Even before Jacob and Monod finally described their view of gene function in 1961, their work had led to an important discovery. On Good Friday in 1960, a small group of researchers, including Crick and Jacob, gathered in Sydney Brenner’s rooms in King’s College, Cambridge, as a kind of ‘after’ meeting following a conference in London on the previous day. Although the Cambridge and Paris groups were on very friendly terms, they were not on precisely the same wavelength. As Brenner later recalled, ‘You see, the Paris people were interested in regulation. We essentially were interested in the code. So we had a slightly different approach.’
41
But that day, the two approaches suddenly fused as Jacob explained the latest results from Paris, focusing on the puzzle of how the z+ gene that enabled bacteria to produce β-galactosidase was able to synthesise such high levels of the enzyme so soon after it was introduced into a
z
–
cell. One of the possibilities that the Paris group had briefly considered was that the gene synthesised a handful of very efficient ribosomes, which then churned out the enzyme at a high rate. But, as Jacob explained, Pardee had recently done an experiment that suggested that the z+ gene did not produce anything stable, but only a rather transitory messenger molecule.
‘At this point,’ recalled Crick, ‘Brenner let out a loud yelp – he had seen the answer.’
42
Jacob vividly described the following minutes:
Francis and Sydney leaped to their feet. Began to gesticulate. To argue at top speed in great agitation. A red-faced Francis. A Sydney with bristling eyebrows. The two talked at once, all but shouting. Each trying to anticipate the other. To explain to the other what had suddenly come to mind. All this at a clip that left my English far behind.