To Tuck, the pinch method seemed an especially promising way to build a fusion reactor. If you could pump enough current into a plasma of deuterium and tritium, the plasma would heat and compress itself all in one fell swoop—perhaps enough to ignite fusion on a small scale. In 1951, Tuck asked for money to build a pinch machine, and in 1952 he built his first. In contrast to Spitzer’s hubristic name—Stellarator implied a mini-sun—Tuck called his instrument the Perhapsatron.
The Perhapsatron, the Stellarator, and the magnetic mirror all showed great promise. At least on paper, they were all able to contain a plasma in a magnetic bottle. Within a few months, scientists had come up with not one but three containers for an uncontainable substance. The Atomic Energy Commission decided to pursue them all. By the time Richter was finally unmasked as a fraud, the United States had consolidated these three efforts into one project: Project Sherwood.
35
At first, Sherwood’s funding was modest, a few hundred thousand dollars or so per year. The budgets would not stay small for long. Though Project Sherwood was classified, it would soon hit the world stage. By mid-1955, rumors abounded that Britain, the USSR, and the United States were all trying to solve the world’s energy problems with fusion—and that U.S. scientists were about to build a prototype fusion reactor. In August, fusion scientists from around the globe met in Geneva for the first UN Conference on the Peaceful Uses of Atomic Energy. The conference president, the Indian physicist Homi J. Bhabha, stunned the world with a bold pronouncement. “I venture to predict that a method will be found for liberating fusion energy in a controlled manner within the next two decades,” he said. “When that happens, the energy problems of the world will truly have been solved forever, for the fuel will be as plentiful as the heavy hydrogen in the oceans.” The dream of fusion energy had been officially made public. Within twenty years, humanity would have limitless energy. The energy problems that had plagued civilization would be a thing of the past.
Lewis Strauss, the head of the AEC, was quick to claim a share of the dream. He confirmed that the United States was hard at work trying to build a reactor that would produce energy. The public and the press began to learn about Project Sherwood, if only the gross details. They knew nothing about the problems that were looming.
Fusion scientists started off very optimistic about their designs; on paper, the machines they were building seemed sure to work. In his 1951 proposal, Spitzer estimated his small Stellarator would generate about 150 million watts of power.
36
The Perhapsatron looked even more promising. It was technically simpler and thus seemed likely to achieve fusion sooner. Once it did, it would be easy to turn a pinch-type device into a reactor. It could behave like a fusion-powered version of an internal-combustion engine: inject fuel, compress it with a current, ignite it, extract the energy, and get rid of the nuclear “ash.” It seemed almost
too
easy, and everybody was pursuing the idea. Despite the secrecy surrounding the early fusion reactor programs, U.S. scientists were certain the British and the Russians were working on pinch-type reactors.
The enthusiasm surrounding the technology, though, hid a lot of difficulties—and some infighting. Spitzer and the Princeton Stellarator team thought their idea was the path to fusion energy, and tried to tear down the Perhapsatron idea championed by their rival, Los Alamos’s Tuck. In fact, Spitzer spent some of his AEC grant trying to prove that a Perhapsatron would not work. It was money well spent. Two of his team members, Princeton professors Martin Schwarzschild and Martin Kruskal, found a very disturbing flaw that threatened to disrupt the Perhapsatron research program altogether. A pinched plasma was unstable.
Perhaps the easiest way to understand stability and instability is to imagine a ball sitting at the bottom of a hill. This is a stable system. Give the ball a slight nudge and it will roll right back to where it started. The system resists change; it won’t be ruined by small perturbations. A ball perched on the top of a steep hill, on the other hand, is in a precarious position. Give it even the slightest nudge and it will roll down the slope, abandoning its previous place. This system doesn’t resist change—indeed, even a tiny disturbance will change it dramatically. This is an unstable system.
Kruskal and Schwarzschild had discovered that a pinched plasma was like a ball perched on a hill. The slightest disturbance would destroy it. Send a current through a cylinder of plasma and it indeed squashes itself into a dense little filament of hot matter. But the filament is unstable. If it is not perfectly straight, if it has even the tiniest kink, the magnetic fields generated by the pinching current immediately exaggerate and expand the kink. This makes the kink grow, getting more and more pronounced. Any little imperfection in the plasma filament rapidly becomes a huge imperfection. In a tiny fraction of a second, the plasma kinks, bends, and writhes out of control.
As soon as the Perhapsatron started up in 1953, the Princeton team’s calculations were proved correct. The Los Alamos experimenters found that as soon as they got a pinch, forming a nice, tight filament in the center of the Perhapsatron’s chamber, it went poof! The pinch would disappear, setting the whole chamber aglow. High-speed cameras revealed the filament buckling and writhing, quickly striking the walls of the chamber. The
kink instability
had claimed its first victim. The Perhapsatron, as built, was incapable of fusing anything at all. The Los Alamos scientists needed to figure out how to stabilize the filament if they were to progress. They tried using an external magnetic field to “stiffen” the plasma filament somewhat, but the essential instability remained. Pinches were in trouble.
KINK INSTABILITY:
If a pinching plasma has even a tiny kink in it, that kink will grow; the plasma will writhe out of control and hit the walls of its container.
Soon, the other designs were as well. In 1954, Edward Teller figured out that a plasma held in place by magnetic fields was unstable under certain conditions. The magnetic fields behave somewhat like a collection of rubber bands: as the plasma pressure increases, they try to relieve the increasing tension by writhing. “They try to snap inward and let the plasma leak out between them,” Teller wrote. This system was also unstable. Even a tiny irregularity in the magnetic field would rapidly get worse, and scientists would lose control of the plasma. The so-called Teller instability affected the Stellarator as well as Livermore’s magnetic mirror approach. Instabilities were everywhere.
By the mid-1950s, all three groups had enormous difficulties to overcome. Their plasmas were unstable and their bottles were leaky. They spent ever-increasing amounts of money building bigger and more elaborate machines in attempts to get unstable plasmas under control. The few hundred thousand dollars spent on magnetic fusion in the early 1950s turned into nearly $5 million by 1955 and more than $10 million by 1957. Plans for reactors also got more ambitious: by 1954, Spitzer was suggesting that $200 million would buy a machine that would produce thousands of megawatts of power—bigger than the biggest power plants around.
Despite Spitzer’s bold plans, Teller’s Livermore got the largest share of funding, about half of the Project Sherwood money. Princeton came in second, and Los Alamos, with its pinch program, was a distant third. Yet it was Los Alamos that first claimed victory.
By the beginning of 1955—just before Bhabha’s speech at the UN conference brought worldwide attention to the promise of fusion energy—the Los Alamos researchers saw indications that their plasma was hot enough to fuse deuterium. Every time they initiated a strong, fast pinch in their latest machine, the scientists saw a burst of tens of thousands of neutrons. This was very encouraging, because neutrons are the best indicator of a fusion reaction.
Everyone in the fusion community was hoping to achieve two main kinds of thermonuclear fusion in a reactor. The easier kind used a mixed fuel: deuterium and tritium. When a deuterium (a proton and a neutron) and a tritium (a proton and two neutrons) strike each other hard enough, they fuse, creating helium-4 (two protons and two neutrons). The remaining neutron flies off with a great deal of energy. So in a successful deuterium-tritium reaction, the products will be helium-4 and neutrons. Deuterium-deuterium reactions are a little more complicated; there are two ways this kind of fusion reaction tends to happen. As the two deuterium nuclei collide and stick, either a proton flies off (leaving behind a tritium nucleus) or a neutron flies off (leaving behind a helium-3 nucleus). These two
branches
of the reaction are roughly equally probable. Thus, if a reactor succeeds in fusing deuterium fuel, then the products will be helium-3, tritium, protons, and neutrons. Neutrons are produced by both deuterium-tritium and deuterium-deuterium fusion reactions. A burst of fusion—no matter whether the fuel is pure deuterium or deuterium mixed with tritium—will be accompanied by a corresponding burst of energetic neutrons.
37
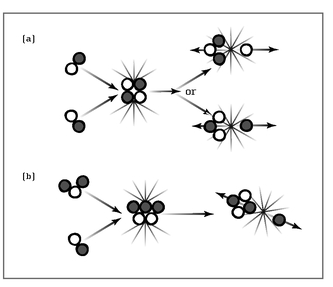
FUSION REACTIONS:
(a) Two deuteriums collide and produce either a tritium and a proton or a helium-3 and a neutron. (b) A deuterium strikes a tritium and produces a helium-4 and a neutron.
Despite the problem with the kink instabilities, Los Alamos scientists were optimistic. If they could make the pinch strong enough and fast enough, they thought, they could get fusion going before the kink instability destroyed the pinch. In fact, Tuck’s calculations showed that such a machine could achieve breakeven—the fusion reaction in the machine would produce energy equal to what was needed to get the reaction going in the first place. (A fusion reactor that absorbs more energy than it produces is of no use to anyone.) And, Tuck argued, a larger machine could produce explosions equivalent to several tons of TNT per pinch. These explosions could be turned into usable energy, just as an internal combustion engine makes little fuel-air explosions turn a crank. Tuck built successively bigger pinch machines that could pinch the plasma harder and faster, and eagerly awaited neutrons produced by thermonuclear fusion.
When, early in 1955, the Los Alamos researchers turned on their newest, biggest pinch machine, Columbus I, they saw a burst of neutrons every time they pinched the plasma hard enough. Pinch. Neutrons. Pinch. Neutrons. No pinch, no neutrons. It seemed like a great success. From the number of neutrons they were seeing, the pinch scientists concluded they had attained fusion; the plasma inside the Columbus machine must have been heated to millions of degrees Celsius. But not everybody was convinced. Researchers at Livermore were skeptical that the pinch machine could reach the temperatures advertised. Thus, the plasma couldn’t possibly be hot enough to ignite a fusion reaction. So where were the neutrons coming from?
The Los Alamos physicists started making careful measurements on their pinch machine to see if they could pin down the origin of those neutrons. To their chagrin, they soon discovered that the neutrons coming out the front of the Columbus machine were more energetic than the ones coming out the rear. In a true thermonuclear reaction, during which nuclei in a hot plasma are fusing with one another, the neutrons from the reaction should be streaming out in all directions with equal energy. This was not the case with Columbus, so, clearly, the Columbus neutrons weren’t coming from thermonuclear fusion. They were coming from somewhere else.
The asymmetry provided a crucial clue. The scientists pinched the plasma by running a current through it. Neutrons that were flying out of the machine in the direction of the current had more energy than those that flew out against it. This revealed that the neutrons were the work of another instability. Just as a pinched filament is unstable when kinked slightly—because the kink grows and grows—it is unstable when a small section gets pinched a little bit more than the rest of the plasma. In this case, the small pinch grows progressively more pronounced; the plasma gets wasp-waisted and pinches itself off. The plasma begins to look like a pair of sausages. This is a
sausage instability
, and it creates some strong electrical fields near the pinch point. These fields accelerate a small handful of nuclei in the direction of the pinch current. These nuclei then strike the relatively chilly cloud of plasma and fuse, releasing neutrons.